Welcome to DU!
The truly grassroots left-of-center political community where regular people, not algorithms, drive the discussions and set the standards.
Join the community:
Create a free account
Support DU (and get rid of ads!):
Become a Star Member
Latest Breaking News
Editorials & Other Articles
General Discussion
The DU Lounge
All Forums
Issue Forums
Culture Forums
Alliance Forums
Region Forums
Support Forums
Help & Search
Environment & Energy
Related: About this forumCalcium Carbonate Decomposition for Cement Clinker and CO2 Removal: Thermodynamics of Relevant Reactions.
The paper to which I'll refer in this post is this one: Calcium Carbonate Decomposition Coupled with In Situ Conversion of CO2 into Syngas: Industrial Scenario Analysis for Carbon Capture and Clinker Production, Peng Jiang, Lin Li, Guanhan Zhao, Tuo Ji, Liwen Mu, Xiaohua Lu, and Jiahua Zhu Industrial & Engineering Chemistry Research 2025 64 (5), 2772-2785.
Before commenting on this paper, I'd like to excerpt another paper to demonstrate the scale at which cement manufacture is driving the extreme global heating we are now experiencing. The excerpt:
The concrete industry exemplifies this, contributing an estimated 6–7% of global anthropogenic (6) and 9–10% of energy related CO2 emissions, (7) with approximately 60% stemming from the calcination reaction inherent to cement production, a process unaffected by renewable energy sources. Consequently, methods for atmospheric CO2 reduction are essential to counteract persistent emissions from hard-to-abate sectors.
The excerpt comes from this paper, which is open to the public: Negative Emission Enabled by Combining Ocean Alkalinity Enhancement and Waste Concrete Upcycling Shijian Jin, Michael D. Tyka, Cristian Rodriguez Martinez, Christopher E. Davis, Christopher Van Arsdale, and Antonio R. Papania-Davis ACS Sustainable Chemistry & Engineering 2025 13 (4), 1532-1543. (The paper refers to a putative "energy transition" for which there is no evidence beyond sloganeering, and also remarks, uselessly, on what so called "renewable energy" is supposed to be able to do, although there is no evidence that it has done a damned thing to even slow the accelerating rate of extreme global heating, but no matter: What is of interest is the estimate of the impact of concrete manufacture.)
The paper cited at the outset is interesting inasmuch as it refers to the decomposition of calcium carbonate in the presence of hydrogen to generate syn gas, thus suggesting a tool for the use of direct heat to eliminate fossil fuel products, assuming of course that the heat is nuclear in origin as is the hydrogen via thermochemical cycling. I am not asserting that this is a practice under development anywhere on Earth - to my knowledge it isn't - but it is a statement of feasibility, not reality. (The reality is that we are doing very little which is useful to address the collapse of the planetary atmosphere.
The paper strikes me as interesting because it has a nice table of the thermodynamics of important carbon reactions that would play a role in carbon dioxide utilization as opposed to horseshit about sequestration, which like "renewable energy will save us" rhetoric, is impractical, dangerous, and useless propaganda to greenwash fossil fuels.
An excerpt from the paper's introduction that I found interesting, as I was unaware of this approach to cement manufacture, which apparently has been known for sometime in theory without any industrial application:
The carbonate industries play an important role in the production of construction materials (such as cement and iron), but the manufacture of them usually involves high temperatures, high energy consumption, and intensive CO2 emissions. (1,2) For instance, the cement industry, one of the largest carbonate industries, has a theoretical energy consumption of 1.67-1.80 GJ for producing one tonne of cement clinker, which mainly contains over 60% CaO, and generates 0.89–1.22 tonnes of CO2. (3) Similarly, in the calcium looping (CaL) process for CO2 capture, the thermal decomposition of CaCO3 theoretically consumes approximately 3.5 GJ of energy per tonne of CaO recycled. (4,5) In 1968, an interesting observation was made regarding the reactions between CaCO3 and pressurized H2, which showed that the decomposition temperature of CaCO3 was reduced by over 100 °C, while simultaneously converting the decomposed CO2 to low-carbon hydrocarbons. (6) Thus, carbonate calcination in H2 atmosphere provides one of the promising strategies to mitigate the challenges of elevated temperatures and CO2-intensive processes faced in carbonate exploitation. (6?9)
The CaCO3 calcination in an H2 atmosphere is considered a coupling reaction of CaCO3 decomposition and the reverse water–gas shift (RWGS). (10,11) In this process, the RWGS utilizes the energy released by CaCO3 decomposition, while removing the CO2 produced from CaCO3 decomposition. (10,11) Compared with CaL combined with CO2 utilization (CCU) technologies, carbonate reductive calcination, as a new integrated CO2 capture and utilization (ICCU) strategy, holds potential for future commercialization. This is due to its ability to avoid the energy and infrastructure costs associated with the capture, compression, and transportation of CO2, which are inevitable in conventional CCU approaches. (12,13) At present, carbonate reductive calcination in H2 atmosphere has attracted widespread interest, with research focusing on optimizing reaction conditions, (14,15) kinetics analysis, (10,16) reaction mechanism, (5,17) and system integration. (17?19) For instance, Shen et al. (15) investigated the CaCO3 hydrogenation with Ni-based catalysts as a promising method for CH4 production. Results indicated that the CaCO3 conversion and CH4 yield reached 89.5% and 80.3%, respectively. Similarly, an in situ CO2 conversion efficiency of nearly 90% and a CO selectivity close to 100% were achieved in the integrated CaL and RWGS process using the heterojunction-redox catalysts of FexCoyMg10CaO. (17) In addition, researchers further analyzed the kinetics of CaCO3 hydrogenation and revealed that the presence of H2 atmosphere increased the CaCO3 decomposition rate by 5-fold compared to Ar. (10) To conclude, the CaCO3 reductive calcination not only alleviates the carbon emissions of the CaCO3 calcination by decreasing the process temperature and increasing the level of CO2 conversion but also obtains high-value gaseous products. So far, about 95% of the H2 is gray H2 and blue H2 in China, the production of such H2 also generates a considerable amount of carbon emissions. (20,21) It is worth noting that CH4 is another hydrogen-donating source and is effective in lowering the decomposition temperature and achieving in situ CO2 conversion. (8,9,22,23) In addition, compared to H2, CH4 could be more advantageous due to its abundance in nature, mature transportation, and storage infrastructure, and most importantly being safer at high calcination temperatures. (24)
The CaCO3 calcination in a CH4 atmosphere can be considered as the coupling reaction of the CaCO3 decomposition and the dry reforming of CH4 (DRM). (25,26) Our previous work investigated a thermodynamic analysis to assess the feasibility of CaCO3 calcination in CH4 (CaDRM). Results indicated that the decomposition temperature of CaCO3 in CH4 was significantly reduced by 170 °C compared to the decomposition in H2, while the CO2 conversion increased from 48.30% in H2 to 72.99% in CH4. (18,22) Though there were benefits of reducing temperature and increasing CO2 conversion, serious carbon deposition was observed, which inhibited further CaCO3 decomposition. (9) This is primarily due to CH4 cracking (CH4 = C + 2H2) in the DRM process. (9,27) The solutions attempting to mitigate carbon deposition in the DRM process were mainly focused on the design of catalyst morphologies and using noble metal catalysts which have so far proven to be cost-prohibitive for commercialization (8,9,25,27)...
The CaCO3 calcination in an H2 atmosphere is considered a coupling reaction of CaCO3 decomposition and the reverse water–gas shift (RWGS). (10,11) In this process, the RWGS utilizes the energy released by CaCO3 decomposition, while removing the CO2 produced from CaCO3 decomposition. (10,11) Compared with CaL combined with CO2 utilization (CCU) technologies, carbonate reductive calcination, as a new integrated CO2 capture and utilization (ICCU) strategy, holds potential for future commercialization. This is due to its ability to avoid the energy and infrastructure costs associated with the capture, compression, and transportation of CO2, which are inevitable in conventional CCU approaches. (12,13) At present, carbonate reductive calcination in H2 atmosphere has attracted widespread interest, with research focusing on optimizing reaction conditions, (14,15) kinetics analysis, (10,16) reaction mechanism, (5,17) and system integration. (17?19) For instance, Shen et al. (15) investigated the CaCO3 hydrogenation with Ni-based catalysts as a promising method for CH4 production. Results indicated that the CaCO3 conversion and CH4 yield reached 89.5% and 80.3%, respectively. Similarly, an in situ CO2 conversion efficiency of nearly 90% and a CO selectivity close to 100% were achieved in the integrated CaL and RWGS process using the heterojunction-redox catalysts of FexCoyMg10CaO. (17) In addition, researchers further analyzed the kinetics of CaCO3 hydrogenation and revealed that the presence of H2 atmosphere increased the CaCO3 decomposition rate by 5-fold compared to Ar. (10) To conclude, the CaCO3 reductive calcination not only alleviates the carbon emissions of the CaCO3 calcination by decreasing the process temperature and increasing the level of CO2 conversion but also obtains high-value gaseous products. So far, about 95% of the H2 is gray H2 and blue H2 in China, the production of such H2 also generates a considerable amount of carbon emissions. (20,21) It is worth noting that CH4 is another hydrogen-donating source and is effective in lowering the decomposition temperature and achieving in situ CO2 conversion. (8,9,22,23) In addition, compared to H2, CH4 could be more advantageous due to its abundance in nature, mature transportation, and storage infrastructure, and most importantly being safer at high calcination temperatures. (24)
The CaCO3 calcination in a CH4 atmosphere can be considered as the coupling reaction of the CaCO3 decomposition and the dry reforming of CH4 (DRM). (25,26) Our previous work investigated a thermodynamic analysis to assess the feasibility of CaCO3 calcination in CH4 (CaDRM). Results indicated that the decomposition temperature of CaCO3 in CH4 was significantly reduced by 170 °C compared to the decomposition in H2, while the CO2 conversion increased from 48.30% in H2 to 72.99% in CH4. (18,22) Though there were benefits of reducing temperature and increasing CO2 conversion, serious carbon deposition was observed, which inhibited further CaCO3 decomposition. (9) This is primarily due to CH4 cracking (CH4 = C + 2H2) in the DRM process. (9,27) The solutions attempting to mitigate carbon deposition in the DRM process were mainly focused on the design of catalyst morphologies and using noble metal catalysts which have so far proven to be cost-prohibitive for commercialization (8,9,25,27)...
Anyway, I always find myself stumbling around in my files and/or the literature for thermodynamic values of key reactions in carbon dioxide utilization, and thus I found this table convenient and quite nearly comprehensive, so it caught my eye:
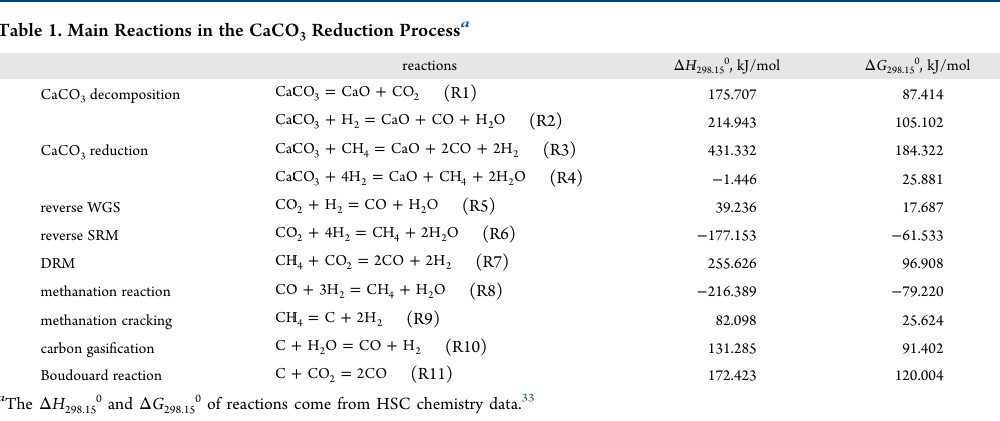
These reactions are key to carbon utilization via the application of primary nuclear heat as an energy source, potentially displacing many of the uses of fossil fuels as convenient, albeit exceedingly dangerous, portable energy sources.
Under the conditions specified in the paper, the effect would be direct air capture of carbon dioxide via the curing of cement, assuming that the syn gas was used either to close the carbon cycle or to utilize carbon as structural or industrial materials.
We are clearly not going to do anything as a species to address extreme global heating, but I merely point out that it would be feasible to do something.